Preface
In the history of the development of contemporary medicine, the 19th century was the golden age of microbial medicine. Scientists laid the foundation for today's microbiological knowledge by discovering microorganisms, cultivating microorganisms and clarifying their significance. They include Antonie van Leeuwenhoek, who discovered microorganisms through a microscope, Robert Koch, who proposed the "Koch's postulates" to establish the causal relationship between microorganisms and diseases, and Louis Pasteur, who clarified the significance of microorganisms, improved vaccinations and invented pasteurization. Early research on microorganisms focused on pathogenic bacteria, and microbiology was often classified as part of the field of infectious medicine. Since Alexander Fleming discovered penicillin in the 20th century, humans have developed various antibiotics over the past century to treat infectious diseases and save many lives. However, under the treatment strategy of eradicating microorganisms, the possibility of coexistence and prosperity between microorganisms and hosts is sometimes overlooked. In fact, there are tens of trillions of bacteria living in the human body, which is more than the number of human cells. The total number of genes contained in intestinal bacteria is 100 times that of human genes. Therefore, the human microbiota can be said to contribute to the human second genome and is even regarded as an important "organ" that has been forgotten in the past. These complex microorganisms include bacteria, fungi and viruses, and are widely present in human skin, respiratory tract, intestinal tract, urinary tract, reproductive tract and other organs. Since the 21st century, scientists have discovered that among the microorganisms distributed throughout the human body, the interaction between the gut microbiota and the host can directly or indirectly affect the host's immune, metabolic and neurotransmission functions. Related research on the human microbiota has opened up a new path for the search for solutions to many chronic and degenerative diseases in humans that had unknown causes in the past. The impact of intestinal bacteria on human health and disease can be discussed from three perspectives: immunity, metabolism, and the gut-brain axis.
Immune system
The immune system is a defense mechanism established by organisms. It mainly rejects abnormal substances in the body, including various microorganisms, parasites, allergens, and tumor cells. Its purpose is to resist the harm caused by foreign substances to the organism. If the human body is likened to a city, the immune system is like a judicial system that is extremely observant, dealing with bad guys while also protecting good guys. For example, training in various environmental stimuli from an early age teaches the immune system to identify friend and foe. On the one hand, it clears pathogens (immune clearance) to control infections and tumors; on the other hand, it establishes immune tolerance with peacefully coexisting environmental antigens (such as symbiotic microorganisms, allergens) to avoid overreactions that lead to allergies and autoimmune diseases.
The intestinal microbiota exists on the digestive tract mucosa, which has a surface area as large as two tennis courts. It is close to the submucosal gut lymphoid tissue (GALT), which is an important site for training the human immune defense system. Whether the intestinal flora can maintain a good state affects the normal development of the host's immune system, is related to the immune system's ability to fight infections and tumors, and may also affect the occurrence of autoimmune diseases. In recent years, researchers from the National Institutes of Health in the United States have discovered that compared to laboratory mice, the intestinal bacteria of wild mice can stimulate the host to produce better immunity to fight influenza viruses and colorectal tumors. The NTU research team also found in animal experiments that the immune regulation mechanism of intestinal bacteria composition will affect the host's ability to clear the hepatitis B virus and is an important factor in whether a person becomes a carrier after an acute hepatitis B virus infection. The team of Kenya Honda from Keio University in Japan further confirmed that the maturation of adaptive immunity, including Th1 helper cells, Th17 helper cells, regulatory T cells (Treg) and cytotoxic T cells, is related to the stimulation of specific intestinal flora. They also found that the related mechanism can affect the severity of inflammatory bowel disease and the ability of the immune system to fight tumors, and even affect the effectiveness of immunotherapy in treating cancer. However, due to the complex interaction between intestinal bacteria and immune cells, the regulatory role played by the intestinal microbiota in the maturation and differentiation of various immune cells in the human body is still not fully understood. If the key molecular mechanisms can be elucidated in the future, it will bring new opportunities for immunotherapy.
Metabolic system
The human body's metabolic system is a collection of biochemical reactions that maintain the normal functioning of life. It covers various organic reactions of decomposition and synthesis, energy use and storage methods, and chemical molecules produced by the metabolic process, as well as the biological reactions, utilization and detoxification processes produced in the body. If the immune system is likened to the judicial system, then the metabolic system is like the financial system, which manages energy storage and consumption. Generally speaking, the body's metabolic capacity changes throughout the life cycle. There are differences in the metabolic capacity of infants, adolescents, adults, the elderly and pregnant women, which is mainly regulated by hormones to cope with developmental and environmental adaptation needs. Even at the same life stage, the metabolic capabilities of different individuals vary significantly. In recent years, many studies have shown that differences in metabolic constitution may come from the regulatory effects of intestinal microbiota and its metabolites on the host, and may be an important factor leading to obesity, diabetes, cardiovascular disease, fatty liver and other diseases. (Figure 1).
The father of intestinal bacteria research, Jeffrey Gordon of Washington University in St. Louis, USA, was the first to discover that under the same genetic background and diet, intestinal bacteria are an important cause of obesity and malnutrition. The effects of short chain fatty acids (SCFAs) produced by intestinal bacteria metabolizing dietary fiber on host cell G-protein coupled receptor 41 and G-protein coupled receptor 43, as well as the downstream signals generated by the interaction of bile acid with farnesoid X receptor and Takeda G-protein coupled receptor, have been proven to be important pathways for intestinal bacteria to regulate host energy expenditure and fat formation. Related research shows that patients with the same genetic background can have different metabolic characteristics, and even people with similar eating habits can have differences in physiques that are prone to obesity or thinness.
In addition to short-chain fatty acids and bile acids, intestinal bacteria also utilize many protein- and lipid-related nutrients in the diet to produce a variety of biologically active chemical products and regulate the host's physiological and metabolic functions. For example, intestinal anaerobic bacteria can use undigested choline and carnitine in food to produce trimethylamine (TMA), which enters the liver and is acted upon by flavin monooxygenase (FMO) to form trimethylamine N-oxide (TMAO) in the human body. After entering the circulatory system, it is excreted by the kidneys. Trimethylamine oxide has been proven to be an independent factor causing atherosclerosis and vascular embolism. It has a dose-dependent effect on the risk of cardiovascular disease and is expected to become an important target for monitoring cardiovascular disease in the future. In addition, some small molecule metabolites that can only be produced by intestinal bacteria have been found to have the potential to affect the host's blood sugar and fat metabolism. For example, indole derivatives, imidazole propionate, phenylacetic acid, etc. are intestinal bacterial metabolites of tryptophan, histidine and phenylalanine, respectively. (Table 1). However, since the relevant microbial metabolic pathways are still not fully understood, which key bacterial species play an important role in affecting the host's physiological mechanisms and which related gene products lead to disease-related metabolic reactions still need to be further clarified in the future.
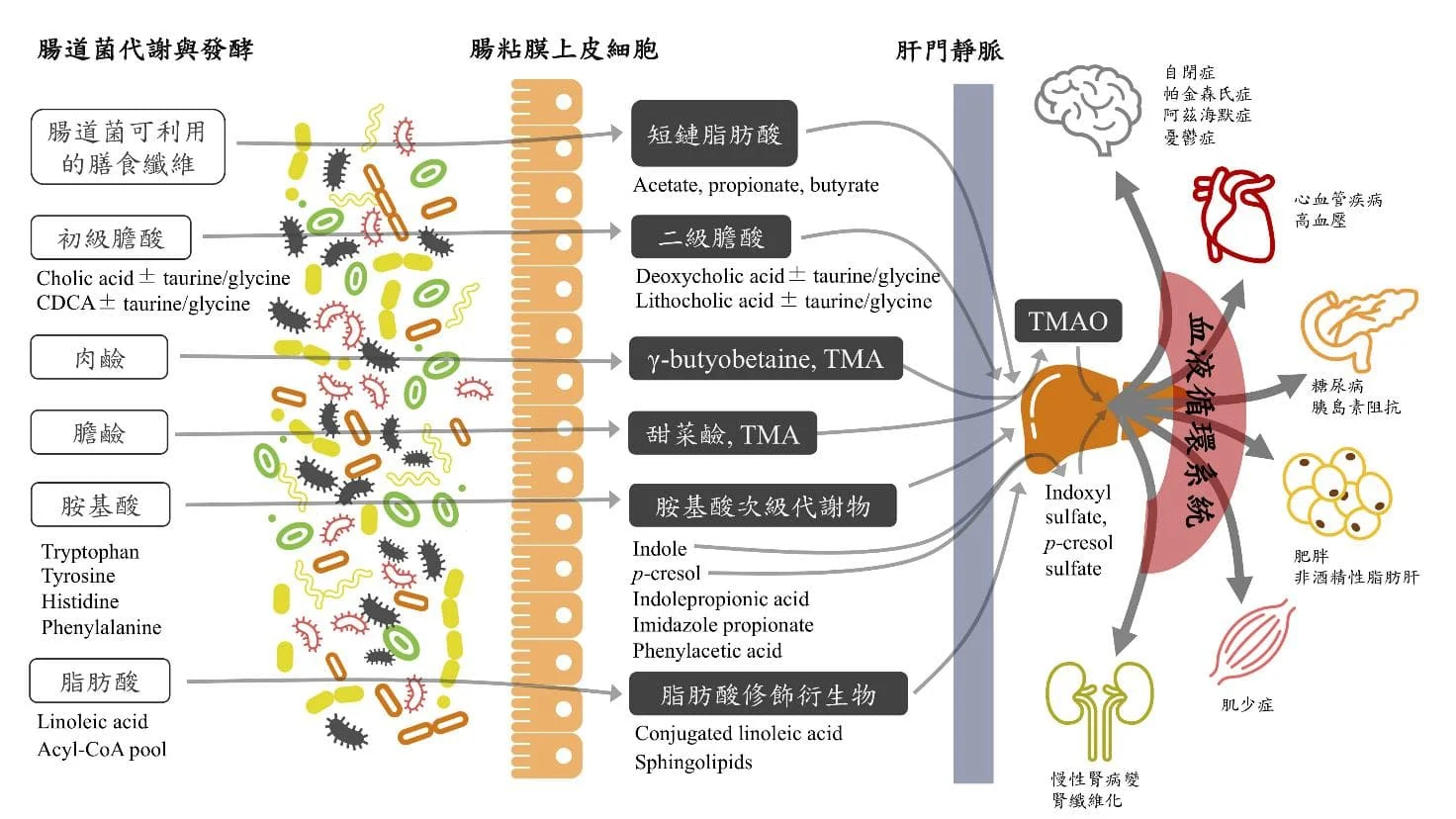
Figure 1. Intestinal bacterial metabolites enter the systemic circulation (gut-systemic axis) from the intestinal cavity and regulate the pathophysiological state of the whole body. (adapted from Wu WK et al. Rapid Commun Mass Spectrom 2020)
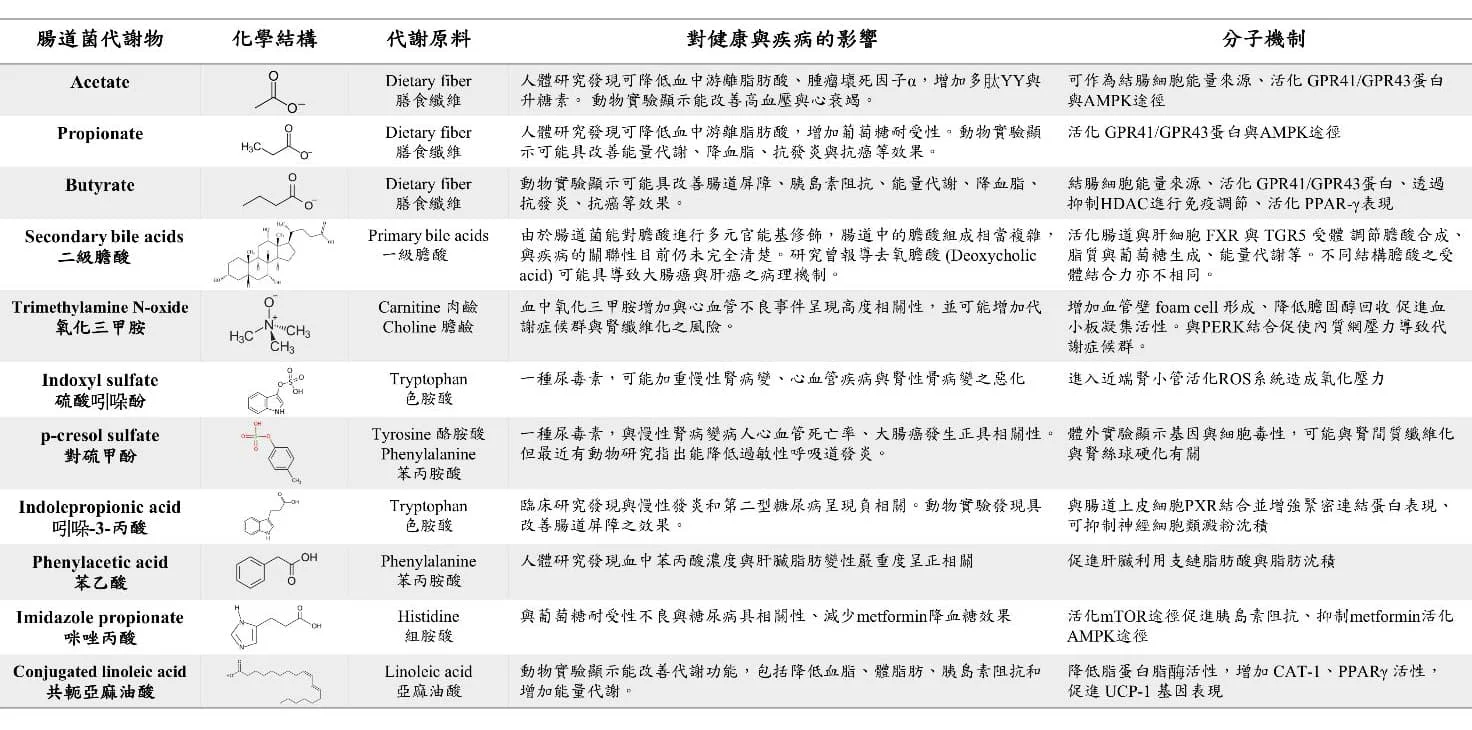
Table 1 Small molecule intestinal metabolites currently known to have biological activity (adapted from Wu WK et al. Rapid Commun Mass Spectrom 2020)
Gut-microbiota-brain axis
In addition to regulating the body's immune and metabolic functions, the intestinal microbiota can also affect the function of the central nervous system (CNS), including developmental (such as autism), degenerative (such as Parkinson's disease, Alzheimer's disease), inflammatory (such as epilepsy) and emotional (such as anxiety, depression) neurological diseases. Even behavioral manifestations such as appetite and eating have been found to be related to the composition and function of intestinal bacteria, and the causal relationship and some mechanisms have been confirmed by research. In fact, the enteric nervous system (ENS) of the human body has the second largest number of neurons and network complexity after the brain. In addition to communicating with the brain through the vagus nerve, it can also operate independently through neurotransmitters secreted by enteroendocrine cells. Therefore, ENS is known as the second brain. Epidemiological studies have found that some degenerative neurological diseases and psychiatric disorders, such as Parkinson's disease, Alzheimer's disease and anxiety disorders, are often accompanied by gut-related comorbidities such as chronic constipation and diarrhea, supporting the close connection between the gut and the brain. At present, the scientific community generally believes that in the gut-microbiota-brain axis, the intestine communicates with the brain mainly through two pathways: one is the downstream signals generated by the interaction of intestinal bacterial metabolites, enteric neurohormones, intestinal submucosal immune response and ENS, which provide information feedback to the brain through the vagus nerve. The other is that intestinal bacterial metabolites and neurotransmitters enter the blood circulation system, pass through the blood-brain barrier (blood brain barrier) into the central nervous system and brain cells to act on them. For example, more than 90% of serotonin (5HT) in the human body is formed by the metabolism of tryptophan in food by enterochromaffin-like cells (ECC) in the intestines, and transmits information to the brain through the vagus nerve. Recent studies have found that the function of ECC to secrete serotonin depends on the stimulation of intestinal bacteria, indicating the regulatory role of intestinal flora in the gut-brain axis. In addition, the human intestinal flora itself can also produce a variety of neurotransmitters in the intestinal cavity, including dopamine, γ-aminobutyric acid (GABA), serotonin, norepinephrine, etc. However, whether these substances can be effectively transmitted to the ENS and CNS and exert their effects still needs further research and confirmation. In summary, although the brain and the intestines are very different organs in terms of anatomical positions, there is indeed an inseparable relationship between the two, and the intestinal microbiota plays a vital role in the communication between the intestines and the brain. The intestines are like the brain's central planning and decision-making tool. If we can elucidate more pathophysiological mechanisms of the gut-flora-brain axis in the future, we will be able to achieve the goal of treating brain diseases with the intestines.
Expert and scholar: Wu Weikai
Recommended Reading
- Allaband C et al: Microbiome 101: Studying, Analyzing, and Interpreting Gut Microbiome Data for Clinicians. Clin Gastroenterol Hepatol 2019; 17:218-30.
- Aron-Wisnewsky et al: Nonalcoholic Fatty Liver Disease: Modulating Gut Microbiota to Improve Severity? Gastroenterology 2020; 158:1881-98.
- Cani PD. Human gut microbiome: hopes, threats and promises. Gut 2018; 67:1716-25.
- Chou HH et al: Age-related immune clearance of hepatitis B virus infection requires the establishment of gut microbiota. Proc Natl Acad Sci 2015; 112:2175-80.
- Chua HH et al: Intestinal dysbiosis featuring abundance of Ruminococcus gnavus associates with allergic diseases in infants. Gastroenterology 2018; 154:154-67.
- Durack J et al: The gut microbiome: Relationships with disease and opportunities for therapy. J Exp Med 2019; 216:20-40.
- Fan Y et al: Gut microbiota in human metabolic health and disease. Nat Rev Microbiol 2021; 19:55-71.
- Gilbert JA et al: Microbiome-wide association studies link dynamic microbial consortia to disease. Nature 2016; 535:94-103.
- Knight R et al: Best practices for analyzing microbiomes. Nat Rev Microbiol 2018; 16:410-22.