How antibiotics make their hosts obese
Antibiotics are the substances that have the most severe impact on intestinal flora. According to a study by Reijnders et al., obese subjects were given 1500 mg of vancomycin and amoxicillin orally for seven consecutive days. It was found that the intestinal flora of the subjects given vancomycin was significantly changed, and the short chain fatty acids (SCFAs) and secondary bile acids (BAs) in the feces also decreased significantly after the administration of vancomycin [1].
In another study, Vrieze et al. gave patients with metabolic syndrome 500 mg of vancomycin and amoxicillin orally for seven consecutive days. After the end of the trial, the subjects who were given vancomycin had significant changes in their intestinal flora, which affected bile acid hydroxylation in the intestine and increased the concentration of primary bile acid in the serum. This in turn reduced the subjects' insulin sensitivity by affecting liver FXR and TGR5[2].
The above literature points out that antibiotics may cause host obesity due to the fact that antibiotics change the metabolites of intestinal bacteria - short-chain fatty acids and bile acids. SCFA can regulate the metabolism of carbohydrates and fats in these tissues through the receptors GPR41/43 (G-protein receptor 41/43) distributed in the liver, adipose tissue, and muscle tissue [3-7]. GPR41/43 distributed in the intestine can promote the secretion of intestinal secretin PYY (peptide YY) and GLP-1 (glucagon-like peptide-1) after receiving the message from SCFA, thereby achieving the effect of suppressing appetite [8-9]. Bile acid has several receptors. The synthesis and excretion of bile acid in the liver are mainly regulated by FXR[10-11]. FXR can also enhance the expression of peroxisome proliferator-activated receptor (PPAR)-alpha and promote fat metabolism in the liver[12]. Transmembrane G protein-coupled receptor (TGR5) is another important bile acid receptor. TGR5 is distributed in brown adipose tissue and muscle tissue. It can promote energy metabolism by regulating type II iodothyronine deiodinase in brown adipose and muscle to increase the concentration of activated thyroid hormone tri-idothyronine (T3)[13].
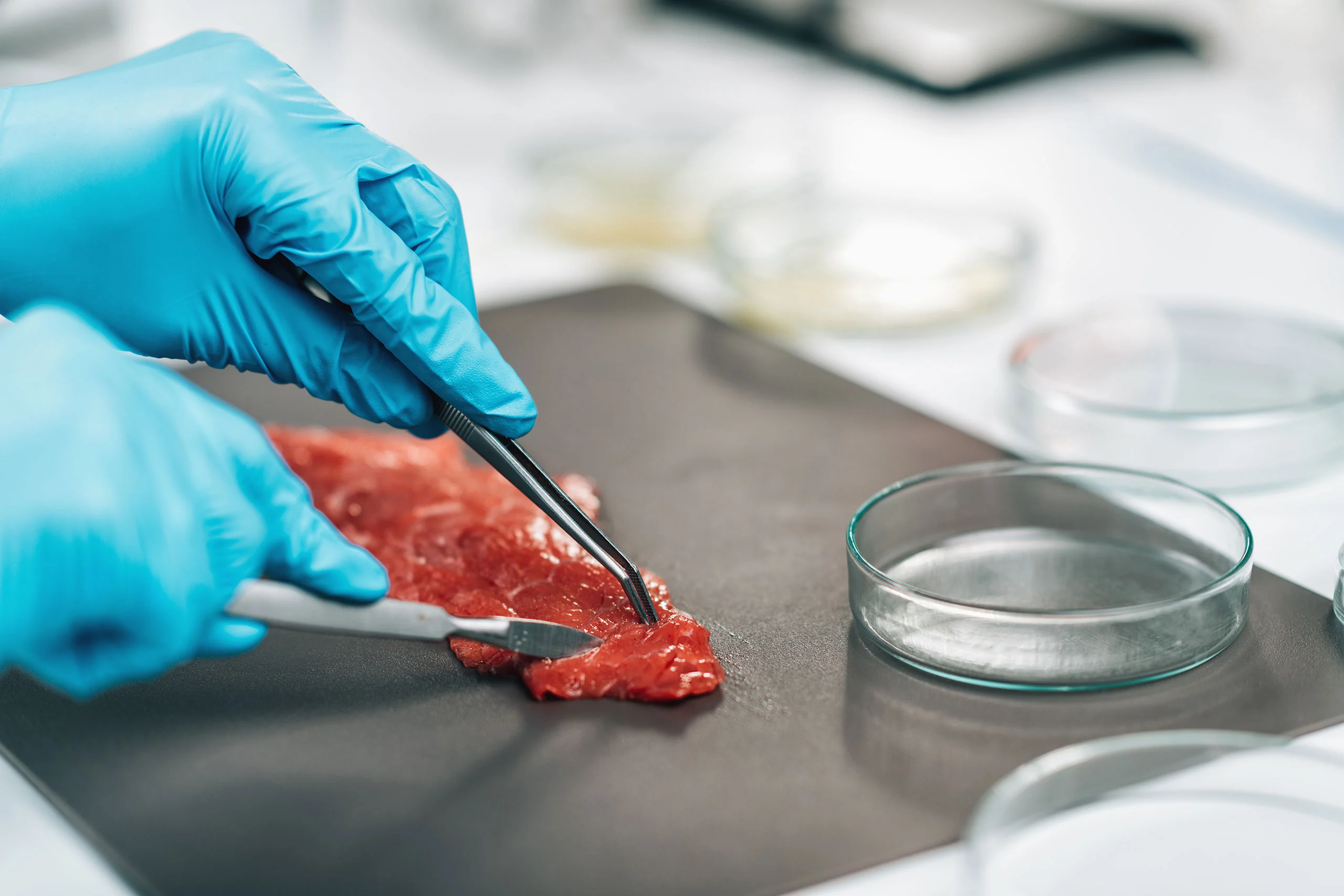
Antibiotics have long been used as growth promoters in the livestock industry
As early as the 1950s, the growth-promoting effect of antibiotics had been discovered and widely used in animal husbandry. Antibiotic growth promoters (AGPs) are government-approved feed additives for non-therapeutic use in animal husbandry. They are usually administered at sub-therapeutic doses to improve feed utilization and promote livestock growth rate[14]. In the past, antibiotics were believed to have a growth-promoting effect based on the fact that they reduce the intestinal bacterial population of the host, thereby reducing the competition for nutrients between intestinal microorganisms and the host, and preventing livestock from growing poorly due to illness[15]. Because antibiotics bring considerable economic benefits to the livestock industry, according to statistics from the U.S. Department of Agriculture in 2011, the amount of antibiotics sold to the meat manufacturing industry in the United States from 2001 to 2011 was three times the amount sold to medical institutions to treat patients, and the trend has been increasing year by year. Domestic animal antibiotics are based on the Guidelines for the Use of Animal Drugs. Article 4, “Standards for the Use of Medicated Feed Additives”, allows 9 antimicrobial agents (Apramycin, Avilamycin, Bicozamycin, Enramycin, Flavomycin, Nosiheptide, Sulfamethazine, Tiamulin, Tylosin) and 17 anticoccidial agents (Amprolium, Clopidol, Cyromazine, Decoquinate, Diclazuril, Ethopabate, Lisalocid, Maduramicin, Monensin, Narasin, Nicarbazin, Ormethoprim, Salinomycin, Semduramicin, Sulfadimethoxine, Sulfaquinoxaline, Zoalene) to be used by feed suppliers or livestock farmers without a veterinarian’s prescription. This means that livestock farmers are allowed to add antibiotics to livestock feed as growth promoters.[16]
Subtherapeutic antibiotic doses affect host metabolism
Recent studies on subtherapeutic doses of antibiotics have provided clear scientific evidence to explain the mechanism by which antibiotics promote animal growth. According to a study published by Cho et al. in Nature, subtherapeutic antibiotic treatment (STAT: penicillin, vancomycin, penicillin+vancomycin, chlortetracycline) was given to weaned C57BL/6J mice for seven weeks. It was found that all four groups of antibiotics increased body fat and serum glucose dependent insulinotropic polypeptide (GIP) levels after intervention. The oral glucose challenge test (OGTT) showed that STAT mice had a tendency to hyperglycemia. According to the analysis of SCFA in mouse feces, the SCFA in the STAT group mice increased, while the analysis of genes related to fat metabolism in the liver found that the expression of genes related to triglyceride (TG) synthesis was increased. This means that SCFA may be transported to the liver through the portal vein, regulating the expression of liver genes to promote fat synthesis, thereby increasing the body fat of mice[17]. Another study published in Cell by Cox et al. further explored whether early-life intervention with subtherapeutic doses of antibiotics (low-dose penicillin, LDP) had an additive effect on obesity caused by a high-fat diet. The results showed that compared with mice fed a high-fat diet alone, mice fed a high-fat diet plus LDP had significantly increased body fat. The introduction of LDP further aggravated the fatty infiltration of the liver caused by the high-fat diet and significantly changed the expression of genes related to liver metabolism and immunity. In addition, ileum gene analysis results showed that LDP also reduced the expression of antimicrobial peptides and T cells in the intestine. To confirm whether intestinal bacteria are the main factor causing metabolic and immune dysfunction in LDP mice, the authors administered feces of LDP mice and control mice to germ-free mice (germ free receptor) by fecal microbiota transplantation (FMT). After FMT, the fecal microbiota composition of the germ free receptor recipients of LDP mouse feces was not only nearly similar to that of LDP mice, but their body fat also increased significantly, and the expression of intestinal immune-related genes also tended to be suppressed. This result confirms that the intestinal flora affected by subtherapeutic doses of antibiotics is an independent factor regulating host metabolism and immune response[18].
International regulations on food additives or residues
The control of the use of antibiotic growth promoters in the livestock industry at home and abroad is mostly in the supply chain from the slaughter of livestock to the sale to consumers in the market, by setting the maximum allowable residue limit (MRL) to control the safety of meat products. The MRL is calculated by dividing the highest no-observed adverse effect level (NOAEL) obtained in toxicology experiments on experimental animals by a safety factor of 100 (10X10= individual difference X species difference) to obtain the acceptable daily intake (ADI). Based on the results of national dietary surveys in various countries, the total amount of a substance that each person may eat from various foods in the diet every day is estimated to calculate the acceptable content of a substance in food, which serves as the standard for the residue limit of a substance in different foods. The theoretical maximum daily intake (TMDI) represents the dietary exposure of a substance. It is calculated by multiplying the MRL of a substance in each food ingredient by the average intake of the food ingredient and then adding the total to get the dietary exposure of the substance. Generally speaking, TMDI must be less than ADI (TMDI is often expressed as % of ADI), which means that the residue of a substance in the diet will not cause harm to humans. However, according to the data of the Joint FAO/WHO Expert Committee on Food Additives (FAO/WHO JECFA), the TMDI of some animal antibiotics is above 80% of ADI, or even exceeds 100% (e.g. TMDI of erythromycin = 130% of ADI) [19]. Furthermore, even if the dietary exposure to a single antibiotic is very low, considering that there are many types of antibiotic residues that may remain in food, whether each antibiotic has an additive effect on intestinal bacteria may require additional experimental design to explore.
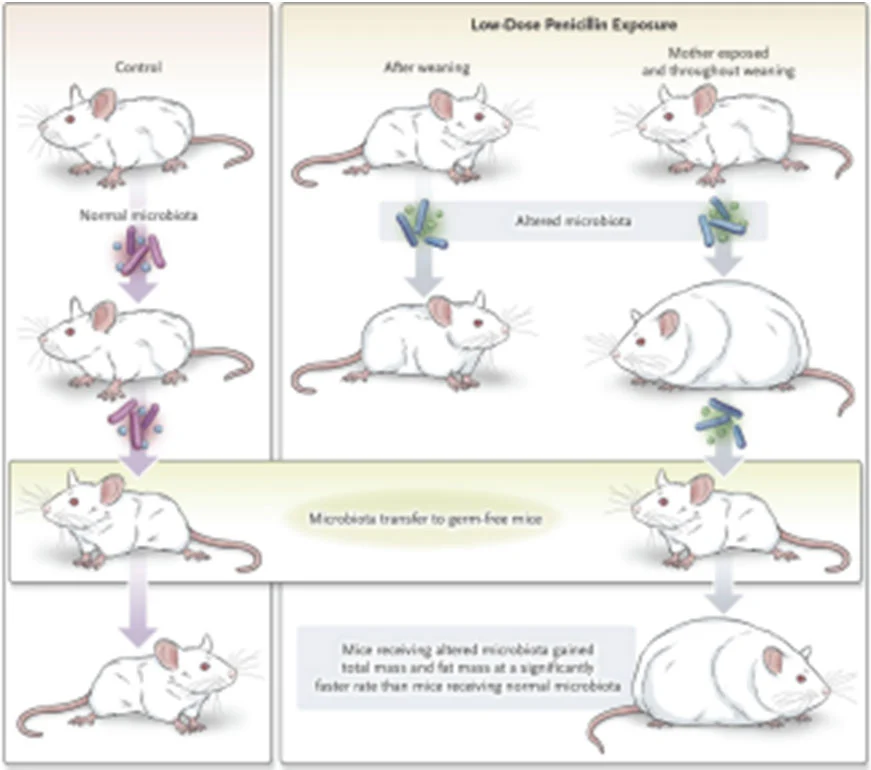
Figure 1 LDP increases body fat in mice by changing their intestinal flora, and this has been verified in a germ-free mouse model.
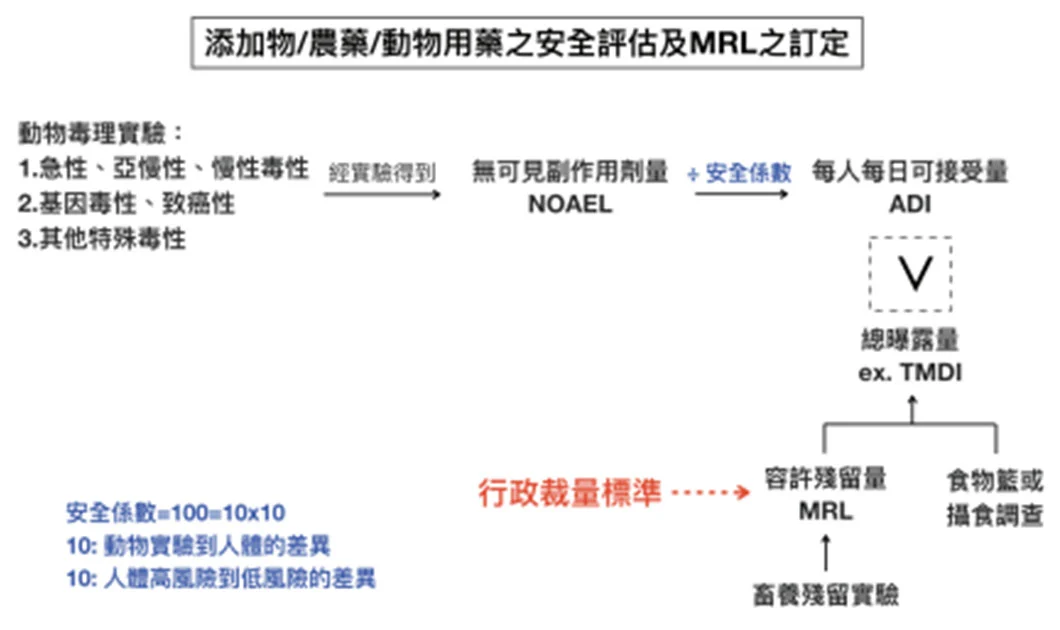
Figure 2 Safety assessment and establishment of limit standards for food additives and residues
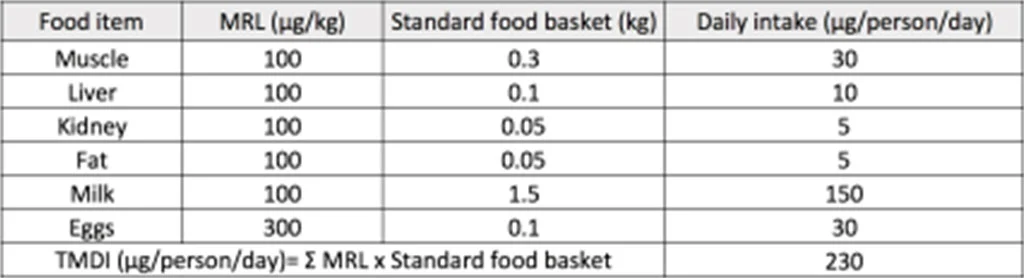
Table 1 Example: TMDI calculation of Tylosin
Potential Food Safety Issues of Antibiotics Used in Animals
In recent years, domestic food safety issues have emerged one after another. How to prevent the occurrence of food safety problems has become an important issue that the government needs to face. In recent years, there have been continuous studies on substances in food that may affect the health of the host, which also means that the basis for food safety risk assessment is not static. Just like the issue of antibiotic use in animals, when people consume meat for a long time, whether the formulation and basis of the limit standards for residual antibiotics in food are sufficient, and whether the definition of so-called "safety" also needs to take into account the occurrence of chronic diseases such as obesity and metabolic syndrome, these issues may need to be re-examined based on changes in epidemiology.
Experts and scholars: Chen Rouan/Lewenhoek Biotechnology Researcher
The original article was published in the 44th Educational Symposium of the Society of Digestive Medicine
References
- Reijnders D, Goossens GH, Hermes GD, et al. Effects of Gut Microbiota Manipulation by Antibiotics on Host Metabolism in Obese Humans: A Randomized Double-Blind Placebo Controlled Trial. Cell Metabolism (2016)
- Anne Vrieze, Carolien Out, Susana Fuentes, et al. Impact of oral vancomycin on gut microbiota, bile acid metabolism, and insulin sensitivity. Journal of Hepatology 2014
- Al-Lahham SH, Roelofsen H, Priebe M, et al. Regulation of adipokine production in human adipose tissue by propionic acid. Eur J Clin Invest 2010 May;40(5):401-7.
- Brown AJ, Goldsworthy SM, Barnes AA, et al. The Orphan G protein coupled receptors GPR41 and GPR43 are activated by propionate and other short chain carboxylic acids. J Biol Chem 2003 Mar 28;278(13):11312-9.
- Fushimi T, Tayama K, Fukaya M, et al. Acetic acid feeding enhances glycogen repletion in liver and skeletal muscle of rats. J Nutr 2001 Jul;131(7):1973-7.
- Bonini JA, Anderson SM, Steiner DF. Molecular cloning and tissue expression of a novel orphan G protein-coupled receptor from rat lung. Biochem. Biochem Biophys Res Commun. 1997 May 8;234(1):190-3.
- Canfora EE, Jocken JW, Blaak EE. Short-chain fatty acids in control of body weight and insulin sensitivity. Nat Rev. Endocrinol. 2015 Oct;11(10):577-91.
- Wichmann A, Allahyar A, Greiner TU, et al. Microbial modulation of energy availability in the colon regulates intestinal transit. Cell Host Microbe 2013 Nov 13;14(5):582-90.
- Lihong Jiang, Barbara Irene Gulanski, Henk M. De Feyter, et al. Increased brain uptake and oxidation of acetate in heavy drinkers. J Clin Invest 2013 Apr 1; 123(4): 1605–1614.
- Goodwin B, Jones SA, Price RR, et al. A regulatory cascade of the nuclear receptors FXR, SHP-1, and LRH-1 represses bile acid biosynthesis. Mol Cell 2000 Sep;6(3):517-26.
- Boyer JL, Trauner M, Mennone A, et al. Upregulation of a basolateral FXR dependent bile acid efflux transporter OSTα- OSTβ in cholestasis in humans and rodents. Am J Physiol Gastrointest Liver Physiol. 2006 Jun;290(6):G1124-30.
- Claudel T1, Staels B, Kuipers F. The Farnesoid X receptor: a molecular link between bile acid and lipid and glucose metabolism. Arterioscler Thromb Vasc Biol 2005 Oct;25(10):2020-30.
- Zietak M, Kozak LP. Bile acids induce uncoupling protein 1-dependent thermogenesis and stimulate energy expenditure at thermoneutrality in mice. Am J Physiol Endocrinol Metab 2016 Mar 1;310(5):E346-54.
- Dibner JJ, Richards JD. Richards. Antibiotic Growth Promoters in Agriculture: History and Mode of Action. Poult Sci 2005 Apr;84(4):634- 43.
- Brown K, Uwiera RRE, Kalmokoff ML, et al. Antimicrobial growth promoteruseinlivestoc k : a requirement to understand their modes of action to develop effective alternatives. Int J Antimicrob Agents 2017 Jan;49(1):12-24.
- Guidelines for the use of animal medicines and regulations for the use of medicated feed additives
- Cho I, Yamanishi S, Cox L, et al. Antibiotics in early life alter the murine colonic microbiome and adiposity. Nature 2012 Aug 30;488(7413):621-6.
- Cox LM, Yamanishi S, Sohn J, et al. Altering the intestinal microbiota during a critical developmental window has lasting metabolic consequences. Cell 2014 Aug 14;158(4):705-721.
- JECFA database (http://apps.who.int/ food-additives-contaminants-jecfa database /Search.aspx)
- Wang H, Wang N, Wang B, et al. Antibiotics detected in urines andadipogenesisinscho ol children. Environ Int. 2016 Apr May;89-90:204-11.
- Wang H, Wang N, Wang B, et al. Antibiotics in Drinking Water in Shanghai and Their Contribution to Antibiotic Exposure of School Children. Environ Sci Technol. 2016 Mar 1;50(5):2692-9.
- Wang H, Ren L, Yu X, et al. Antibiotic residues in meat, milk and aquatic products in Shanghai and human exposure assessment. Food Control 2017 Oct P217-225